Different fishes live in waters from almost distilled purity, to hypersaline ponds where they have difficulty keeping below the surface. The ability to live in such different waters is remarkable enough for stenohaline fish which do not move from a single environment, but, perhaps more striking, there are a good many euryhaline species able to move (sometimes rapidly) from fresh water to the sea and back again. It is obvious that fresh water fish cannot be isosmotic with the external medium, but, less obviously, very few marine are isosmotic with seawater.
Although fish skin is on the whole rather imper-meable (puzzlingly so in those fish where cutaneous respiration is important),the problem is that fish have large areas of permeable epithelium in close con-tact with the water. Not only is there the vast area of the gill epithelium, but there are the narial and oral mucosae , and some water will inevitably be swallowed as the fish feeds. Since very few fishes are isosmotic with the external medium, there will be osmotic gradients across these permeable surfaces, and fish have to devote 25–50% of their total energy output to cope with this. In marine teleosts, these are of the order of 600–800 mosmol kg–1, and water will tend to be lost and ions gained, while, in freshwater fish, water will tend to be gained and ions lost. It may seem surprising that the osmoregula-tory mechanisms for coping with these opposite problems in seawater and freshwater can be switched by euryhaline fish as they move from one to the other.
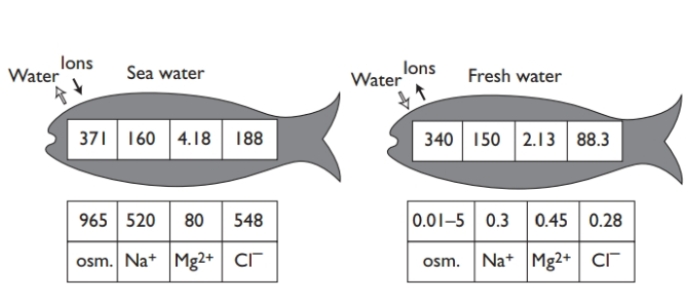
Changes in body fluids indeed occur as euryhaline fish are confronted with salinity changes: thus in eels, salmon, and flounders (Platichthys flesus) plasma osmolarity rises by 20% or so (mainly due to increase in plasma Na+) when they adapt from freshwater to seawater. The little killifish, Fundulus, can surmount the greatest osmotic challenge, living in nature both in freshwater, and in southern California in hypersaline pools (128‰ salinity). Naturally, it has been the subject of much experiment, can reg-ulate body water and plasma salt concentration between 0 and 60% NaCl.
Higher salinities are tolerated by accepting 5% tissue water loss, and an increase in blood osmolarity of around 30%. Recent work has indicated that when killifish enter waters of different salinity, the switch from Cl– secretion on entering low salinity water to Cl– absorption and vice versa is a direct effect on the osmo-sensing chloride cells themselves, causing changes in gene expression. When challenged by transfer from freshwater to seawater, num-bers of chloride cells increase, as has been shown by laser scanning cytometry. Such short term changes following transfer to different salinities may be followed by longer-term changes in gene expression (Scott et al., 2004). In eels, which can cope with rapid changes from freshwater to seawater, natriouretic peptides and the renin-angiotensin hormones are involved (Takei and Hirose, 2002).

The reader will surely already have realized that the large areas of perme-able epithelium in contact with the surrounding water imply that extra renal routes of excretion and ion exchange can be exploited, in contrast to the renal routes of most terrestrial forms, but before considering the mechanisms involved (recently reviewed by Evans et al., 2005), we may first examine the sit-uation in hagfish, unique not only in being isosmotic with seawater, but also in having blood Na+ and Cl– levels similar to seawater.